The Wave of the Century
WSU researcher part of group that discovered evidence for the existence of gravitational
CODY COTTIER | The Daily Evergreen
Scientists use tubes inside these concrete arms to measure fluctuations in the distance between their extremities, caused by gravitational waves.
March 10, 2017
The view from Sukanta Bose’s ninth-floor Webster Hall office looks out over the crests and troughs of wheat hills, autumn-browned waves rolling south.
The scene is striking and I tell him so. “Yes,” he laughs, “I am fortunate to have this office.” But the waves that captivate Bose originate farther beyond his window, far past the Palouse.
Bose, a WSU physics and astronomy professor, was among an international group of researchers who discovered direct evidence for the existence of gravitational waves in September 2015. In the time since, he has spent a great deal of time traveling, lecturing at universities and attending meetings in the U.S. and abroad.
“It’s not good for life in general,” he says, “but sometimes you realize that such opportunities don’t come your way all the time.”
For many years, Bose and other researchers in his field waited for just such an opportunity. The instruments of the time, in their infancy in the late 1990s, were not advanced enough to detect any but the strongest gravitational waves.
“In 1997 it was a leap of faith,” Bose says, “because it wasn’t clear whether or when these observatories would actually find anything.”
But looking back from this side of the discovery, a groundbreaking event that confirmed Einstein’s theory of general relativity on the centennial of its publication, Bose reflects on what the scientific community has gained — a new way of probing the universe that was inaccessible to scientists as long as they were restricted to electromagnetic waves.
“I think it was worth it, because this field had promise,” he says. “It was like a Galileo moment.”
“The biggest glory”
Atop a filing cabinet in Bose’s office sits a can of sardines, labeled with the brand name “Ligo” – an obscure joke to anyone unfamiliar with astrophysics and gravitational wave science. But to Bose, the student who gave him the can and their colleagues, the humor is apparent.
“I have not tasted those,” Bose laughs.
This is because they were not intended for eating. The sardines share a name with the LIGO (Laser Interferometer Gravitational-Wave Observatory) detectors, devices used to measure the ripples in space-time caused by gravitational waves.
About 1.3 billion years ago, two distant, massive black holes spiraled together after orbiting each other for hundreds of millions of years, releasing tremendous amounts of energy in the form of gravitational waves in the final seconds before they merged into each other. When the waves reached Earth in late 2015, they caught the attention of scientists at two detectors: one at the Hanford Site, near Richland, and one in Livingston, Louisiana.
“That morning,” says Vern Sanders, a staff scientist at the Hanford detector, “our perception … of the world changed in a fundamental way.”
Sanders says the ability to use gravitational waves as tools for science will lead to a better understanding of black holes and other exotic cosmic phenomena. He says some considered the discovery worthy of a Nobel prize, but the cutoff date for nominations fell just before they announced it.
“The biggest glory is that we found it,” he says. “This stuff is real.”
Physicists Russell Hulse and Joseph Taylor received the Nobel Prize in 1993 for indirectly discovering gravitational waves. The National Science Foundation approved the construction of the LIGO detectors the next year, two years before Bose would complete his Ph.D. at the University of Wisconsin.
At the time, he says, gravitational waves were a source of both intrigue and uncertainty. The available instruments did little to encourage scientists who thought electromagnetic waves the safer bet, far more visible and easier to work with. But LIGO detectors brought promise, the chance to reexamine the cosmos by methods that had for decades been purely theoretical.
“Interest in gravity for me was always there,” Bose says. “But then there was this tantalizing prospect.”
The problem remained, however, whether there would be sources of gravitational waves near enough to Earth for the detectors to observe. Even then, supposing they did receive signals, the volume of data involved would strain computations.
“It was high-risk, high-gain,” Bose says, “and I chose to take a risk.”
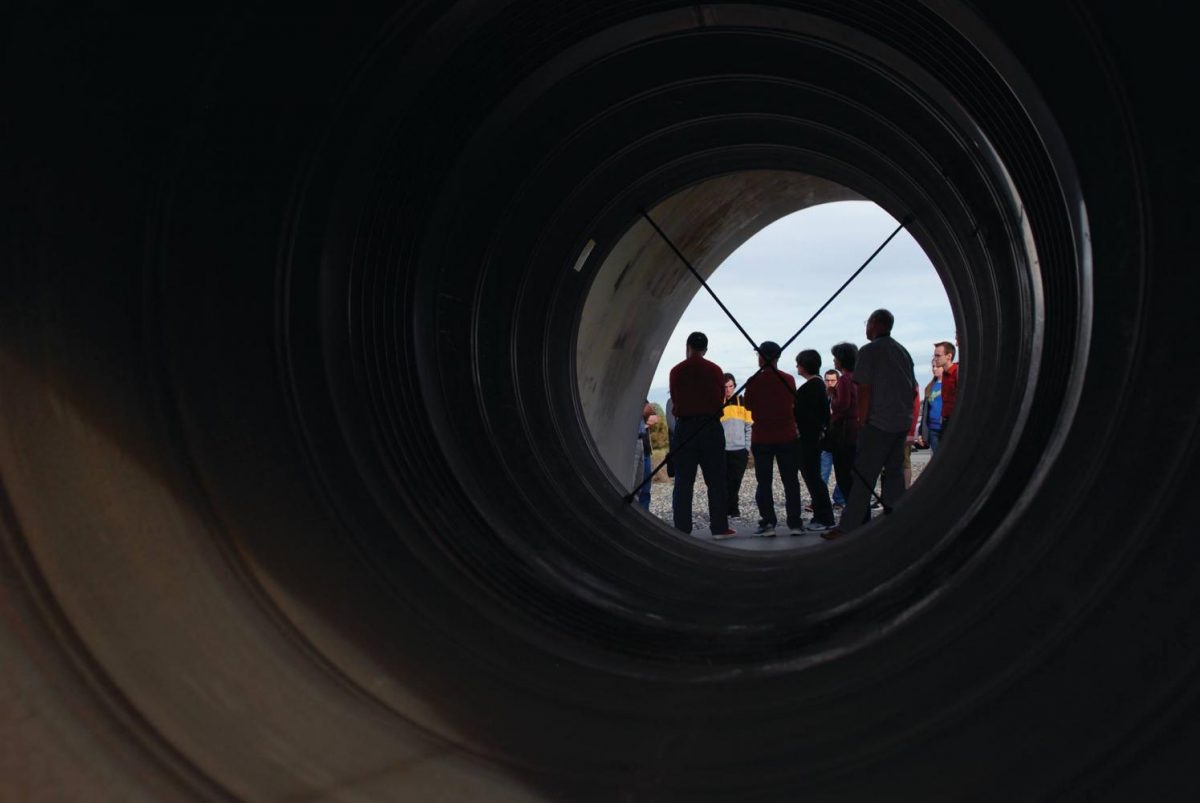
Running the gantlet
Between Sept. 14, 2015, and Feb. 11 of the following year, Bose and hundreds of other scientists had a secret to keep.
It seemed the risk had paid off when the LIGO detectors picked up their first signal in the middle of the night, Hanford time. Bose, halfway around the world in India, was drinking his afternoon cup of tea when he received an email alerting him of “something interesting” logged in the database.
“It did raise the temperatures a bit,” he says. “It was very exciting. But because it was the first one, I have to admit that I was not fully sure that this is real.”
But he was sure it was serious enough to make the effort to determine whether it was real. Over the next several months, he and his colleagues worked to eliminate possible causes of the signal besides gravitational waves, checking hundreds of thousands of monitors used to track “noise” from other sources.
Bernard Hall, a graduate student who has worked with Bose for several years designing software to help with this process, compared the signal to a whisper in a sawmill. The detectors, he says, are sensitive enough to pick up the distant rumble of cars on the highway, and even the ocean waves beating against the continental plates.
“That’s kind of what we’re up against to detect something like that,” Hall says.
But the importance of certainty is paramount. Bose notes the BICEP2 blunder of 2014, in which a group of scientists announced they had discovered gravitational waves by observing their faint imprints on the cosmic background radiation, thermal radiation left over from the Big Bang. Soon after, but not before the scientists received numerous awards for their work, peer review revealed they had actually detected only cosmic dust.
“Of course, the team was directly hit by this,” Bose says, “but science in general also receives some of that negative impact, so we have to be very careful about this.”
During the months between discovery and announcement, Hall would gauge Bose’s excitement level, looking for signs of hope. Hall says Bose foresaw the BICEP2 mistake, realizing the researchers had not accounted for dust, and this was reflected in his low interest in the subject.
“But it was different with [our discovery],” he says. “I was kind of more like ‘It’s probably just a glitch or something like that,’ but he kept talking about it and I was like ‘Really? OK.’”
Nevertheless, they tested alternatives until they were satisfied the discovery was legitimate. Their standard is the five sigma threshold, where the higher the sigma value, the more likely they have detected a genuine signal. Bose says each time a discovery reached that threshold in the past, future investigation validated it, assuring scientists of its accuracy.
“Once every 200,000 years, noise can do this,” Bose says. “That’s extremely unlikely. That’s like flipping a coin 22 times and every time consistently getting a head.’”
It takes a certain kind of machine to reach such reliable results.
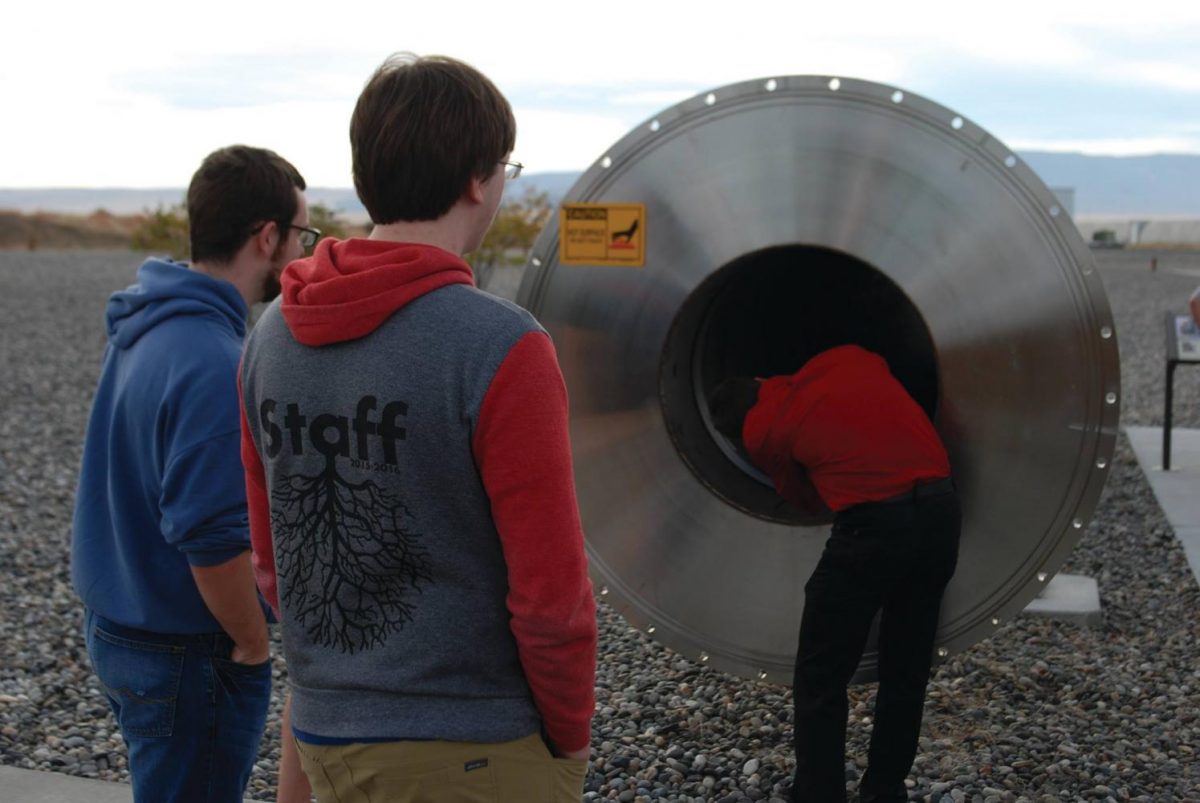
WSU students with the Physics and Astronomy clubexamine a sample of the tube used in LIGO detectors
Something tangible
Two half-submerged concrete arms stretch four kilometers out from the Hanford LIGO Observatory, disappearing from sight into the sagebrush-strewn desert. Bose occasionally visited the observatory to confer with colleagues, and the student and postdoctoral researchers would sometimes accompany him. Besides seeing the physical applications of his work, Hall recalls running up and down the massive arms, which house the tubes used to measure gravitational wave disturbances.
“A lot of the stuff I do is just remotely by logging into remote computers and stuff like that, which is fine and good,” he says. “But actually being able to go there and see, it’s more than just abstract. There’s this actual, tangible thing.”
We hear often nowadays of nanotechnology. A nanometer is the billionth part of a meter, and a million protons stacked up will be a nanometer tall. At this scale we are approaching the lengths LIGO detectors measure during gravitational wave disturbances, but we are not quite there.
“This is even smaller,” Bose says. “Take a proton and divide it into 1,000 to 10,000 pieces, and then what you get is the change in length that a highly energetic cosmic event would produce in a detector.”
To begin, the instrument shoots a laser at a light splitter, which redirects the beam down the two tubes. At the end of each tube sits a mirror. Absent gravitational disturbance, the beams bounce off these mirrors and reflect back to the light splitter, where, due to their relative phase difference, they cancel each other out.
When a wave comes along, it distorts the space between the light splitter and the mirror, causing the two beams to fall out of phase. When this happens, light lands upon a photodetector, signaling the scientists to inquire further.
“If you talk to an experimenter,” Bose says, “they say … ‘Gravitational waves are great,’ but they are on a high just because of the fact that they have produced an instrument that can measure gravitational waves.”
The next phase
The instrument is surely a feat. But science advances in a perpetual state of discontent, the unknown fueling progress, and the LIGO detectors seem to follow suit. Their first observations ran from 2002 to 2010, when they were shut down for upgrades. They resumed operations in 2014, and soon after made their first detection. The LIGO Scientific Collaboration announced a second detection in June, 2015, and as of December, 2016, the detectors are under a second, phased renovation with the aim of increasing their sensitivity. Meanwhile, additional detectors are in the works in Italy and Japan.
A site has also been selected for another detector in India, and in the coming years it will join the rest to aid in more precise measurements. Bose, who was born in Kolkata, India, was heavily involved in obtaining funding and approval for this project. His work is split between Washington and India, and he has traveled more extensively since the discovery was announced, though his trips are primarily consumed by work.
“Travel is painful,” he says. “It may sound like ‘Oh, wow, you are spending a week in Paris.’ [But] believe me, I have not seen much of Paris and I’ve been there three times.”
His wife Smitha and their daughter Symanthika, 15, and son Svanik, 8, join him on his travels when they are able. The rest of their time is spent in Pullman, where the children attend school and Smitha works in WSU’s Office of International Programs. Svanik sometimes visits his father’s office, as evidenced by the Mickey Mouse drawn on Bose’s whiteboard amid scribbled calculations.
His work demands a great deal. Though there was a time when the future of his field of work seemed uncertain, and though in some ways it still is, the recent advancements in gravitational wave research have already begun to pay off on Bose’s investment.
“It costs you in terms of your health and the time you can give to your family,” he says, “but I know that this phase — every phase — has a shelf life, and it can last only this long. And if you don’t cash on it, someone else will.”